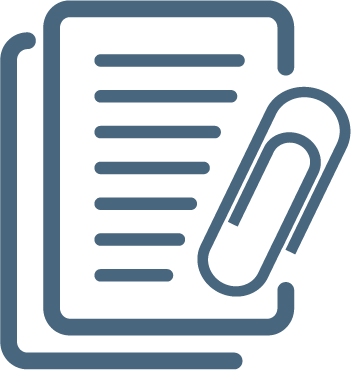
Journal of Fish Biology
SCOPUS (1969-2023)SCIE-ISI
0022-1112
1095-8649
Anh Quốc
Cơ quản chủ quản: WILEY , Wiley-Blackwell Publishing Ltd
Các bài báo tiêu biểu
Methods for analysing fish stomach contents are listed and critically assessed with a view to their suitability for determining dietary importance—this term is defined. Difficulties in the application of these methods are discussed and, where appropriate, alternative approaches proposed. Modifications which have practical value are also considered. The necessity of linking measurements of dietary importance to stomach capacity is emphasized and the effects of differential digestion upon interpretation of stomach contents outlined. The best measure of dietary importance is proposed as one where both the amount and bulk of a food category are recorded.
A survey of the studies published in two leading fisheries journals revealed that the analysis and measurement of condition, based on length‐weight data, has been performed using a wide variety of indices and statistical procedures. Eight forms of index were identified which can be categorized into those which measure the condition of individual fish, i.e. condition factors‘, and those which measure the condition of subpopulations as a whole, i.e. regressions of log, 10 weight on log, 10 length and the parameters of such regressions. Analysis of a test data set indicated that both the form of index and properties of the data set size can dictate the patterns of condition observed. The various indices were reviewed in terms of appropriateness, simplicity and statistical correctness. It was concluded that an index should be selected only after a detailed examination of both the underlying assumptions of the index and the properties of the data set.
FISH‐BOL, the Fish Barcode of Life campaign, is an international research collaboration that is assembling a standardized reference DNA sequence library for all fishes. Analysis is targeting a 648 base pair region of the mitochondrial cytochrome
Ongoing climate change is predicted to affect individual organisms during all life stages, thereby affecting populations of a species, communities and the functioning of ecosystems. These effects of climate change can be direct, through changing water temperatures and associated phenologies, the lengths and frequency of hypoxia events, through ongoing ocean acidification trends or through shifts in hydrodynamics and in sea level. In some cases, climate interactions with a species will also, or mostly, be indirect and mediated through direct effects on key prey species which change the composition and dynamic coupling of food webs. Thus, the implications of climate change for marine fish populations can be seen to result from phenomena at four interlinked levels of biological organization: (1) organismal‐level physiological changes will occur in response to changing environmental variables such as temperature, dissolved oxygen and ocean carbon dioxide levels. An integrated view of relevant effects, adaptation processes and tolerance limits is provided by the concept of oxygen and capacity‐limited thermal tolerance (OCLT). (2) Individual‐level behavioural changes may occur such as the avoidance of unfavourable conditions and, if possible, movement into suitable areas. (3) Population‐level changes may be observed
Human beings may affect the welfare of fish through fisheries, aquaculture and a number of other activities. There is no agreement on just how to weigh the concern for welfare of fish against the human interests involved, but ethical frameworks exist that suggest how this might be approached.
Different definitions of animal welfare focus on an animal's condition, on its subjective experience of that condition and/or on whether it can lead a natural life. These provide different, legitimate, perspectives, but the approach taken in this paper is to focus on welfare as the absence of suffering.
An unresolved and controversial issue in discussions about animal welfare is whether non‐human animals exposed to adverse experiences such as physical injury or confinement experience what humans would call suffering. The neocortex, which in humans is an important part of the neural mechanism that generates the subjective experience of suffering, is lacking in fish and non‐mammalian animals, and it has been argued that its absence in fish indicates that fish cannot suffer. A strong alternative view, however, is that complex animals with sophisticated behaviour, such as fish, probably have the capacity for suffering, though this may be different in degree and kind from the human experience of this state.
Recent empirical studies support this view and show that painful stimuli are, at least, strongly aversive to fish. Consequently, injury or experience of other harmful conditions is a cause for concern in terms of welfare of individual fish. There is also growing evidence that fish can experience fear‐like states and that they avoid situations in which they have experienced adverse conditions.
Human activities that potentially compromise fish welfare include anthropogenic changes to the environment, commercial fisheries, recreational angling, aquaculture, ornamental fish keeping and scientific research. The resulting harm to fish welfare is a cost that must be minimized and weighed against the benefits of the activity concerned.
Wild fish naturally experience a variety of adverse conditions, from attack by predators or conspecifics to starvation or exposure to poor environmental conditions. This does not make it acceptable for humans to impose such conditions on fish, but it does suggest that fish will have mechanisms to cope with these conditions and reminds us that pain responses are in some cases adaptive (for example, suppressing feeding when injured).
In common with all vertebrates, fish respond to environmental challenges with a series of adaptive neuro‐endocrine adjustments that are collectively termed the stress response. These in turn induce reversible metabolic and behavioural changes that make the fish better able to overcome or avoid the challenge and are undoubtedly beneficial, in the short‐term at least.
In contrast, prolonged activation of the stress response is damaging and leads to immuno‐suppression, reduced growth and reproductive dysfunction. Indicators associated with the response to chronic stress (physiological endpoints, disease status and behaviour) provide a potential source of information on the welfare status of a fish. The most reliable assessment of well‐being will be obtained by examining a range of informative measures and statistical techniques are available that enable several such measures to be combined objectively.
A growing body of evidence tells us that many human activities can harm fish welfare, but that the effects depend on the species and life‐history stage concerned and are also context‐dependent. For example, in aquaculture, adverse effects related to stocking density may be eliminated if good water quality is maintained. At low densities, bad water quality may be less likely to arise whereas social interactions may cause greater welfare problems.
A number of key differences between fish and birds and mammals have important implications for their welfare. Fish do not need to fuel a high body temperature, so the effects of food deprivation on welfare are not so marked. For species that live naturally in large shoals, low rather than high densities may be harmful. On the other hand, fish are in intimate contact with their environment through the huge surface area of their gills, so they are vulnerable to poor water quality and water borne pollutants.
Extrapolation between taxa is dangerous and general frameworks for ensuring welfare in other vertebrate animals need to be modified before they can be usefully applied to fish.
The scientific study of fish welfare is at an early stage compared with work on other vertebrates and a great deal of what we need to know is yet to be discovered. It is clearly the case that fish, though different from birds and mammals, however, are sophisticated animals, far removed from unfeeling creatures with a 15 s memory of popular misconception. A heightened appreciation of these points in those who exploit fish and in those who seek to protect them would go a long way towards improving fish welfare.
From a total of 524 microsatellite loci considered in nearly 40 000 individuals of 78 species, freshwater fish displayed levels of population genetic variation (mean heterozygosity, h=0·46, and mean numbers of alleles per locus, a=7·5) roughly similar to those of non‐piscine animals (h=0·58 and a=7·1). In contrast, local population samples of marine fish displayed on average significantly higher heterozygosities (h=0·79) and nearly three times the number of alleles per locus (a=20·6). Anadromous fish were intermediate to marine and freshwater fish (h=0·68 and a=11·3). Results parallel earlier comparative summaries of allozyme variation in marine, anadromous, and freshwater fishes and probably are attributable in part to differences in evolutionarily effective population sizes typifying species inhabiting these realms.
We present the first synthesis of the life history and ecology of seahorses, compare relationships for seahorses with other marine teleosts and identify research needs. Seahorses occurred primarily amidst temperate seagrasses and tropical coral reefs. Population densities were generally low, ranging from 0 to 0·51 individuals m−2, but reached 10 m−2in some patches. Inferred life spans ranged from 1 to 5 years. Seahorses consumed live prey and possibly changed diet as they grew. Growth rates are poorly investigated to date. Reproduction and mating systems are the best‐studied aspects of seahorse ecology. The relationship between size at first maturity and maximum size in seahorses conformed to that for other marine teleosts. All seahorse species were monogamous within a cycle, but some were polygamous across cycles. Direct transfer of clutches to the brood pouch of the male fish made it difficult to measure clutch size in live seahorses. After brooding, males released from
Empirical relationships are presented to estimate in fishes, asymptotic length (L∞) from maximum observed length (Lmax), length at first maturity (Lm) from L∞, life span (tmax) from age at first maturity (tm), and length at maximum possible yield per recruit (Lopt) from L∞ and from Lm, respectively. The age at Lopt is found to be a good indicator of generation time in fishes. A spreadsheet containing the various equations can be downloaded from the Internet at
The round goby
The response of the brown trout to a single, short (